Magnetic Shielding
Experiments with magnets and our surroundings
- Magnetic Shielding
- Magnetic Shielding Box
- Magnetic Shielding Bag
- Magnetic Shielding Metal
- Magnetic Shielding Material
- Magnetic Shielding Material
Magnetic shielding ensures that these sensitive pieces of equipment operate properly. While the strategies are different, we are able to shield both AC and DC magnetic interference using specially processed magnetic shielding materials.
How can you shield a magnet?
- Magnetic shielding of this type does not guard against high frequency electromagnetic fields outside the scanner, however. This requires a different type of protection, called radiofrequency (RF) shielding. RF shielding methods will be described in several follow-up questions.
- Home Magnetic Shielding Magnetic Shielding Materials We select magnetic shielding materials for their specific characteristics, usually with respect to permeability and saturation. Permeability determines the effectiveness with which a given shield can.
Have you ever wondered how you can shield the magnetic field created by a magnet?
Is there any material that you can place between two magnets so they would not affect each other?
The answer is, 'Yes, to an extent!'
Two Magnets, Attraction
Suppose you have two magnets, spaced a certain amount apart, and the North poles are both facing the same direction so that the South pole of one is facing the North pole of the other. This is shown in the diagram on the left. The North poles for both magnets are to the right. These magnets are attracted to each other.
The diagram in the middle shows what happens if you insert an iron plate between the magnets. No change? Actually, each magnet is now attracted to the iron plate, and don't see the other magnet on the other side of the plate!
The diagram on the right shows what happens if you insert a superconductor between the magnets. Now, each magnet is repelled by the superconductor, and there is little or no attraction between the magnets themselves (one little field line did sneak around the superconducting plate, though).
Two Magnets, Repulsion
Suppose you have two magnets, spaced a certain amount apart, and the North poles are both facing each other. This is shown in the diagram on the left. These magnets are repelling each other.
The diagram in the middle shows what happens if you insert an iron plate between the magnets. This is kind of like the middle diagram in the group above this, isn't it? This time, each magnet is now attracted to the iron plate, and don't see the other magnet on the other side of the plate, even though both of the North poles are facing each other!
The diagram on the right shows what happens if you insert a superconductor between the magnets. Very little change from no plate! Each magnet is repelled by the superconductor, and there is no repulsion between the magnets themselves.
One Magnet
Suppose you have one magnet. Now what happens? The diagram on the left shows the field created by that one magnet.
The diagram in the middle shows what happens when you place an iron plate near that magnet. The field on the other side of the plate is almost nil. This is because the plate has diverted the field, causing a lot of it to flow within the plate itself instead of in the air. To see this in action, see Magnets in Motion 10 and Magnets in Motion 11. This is sometimes used to help shield a speaker from your TV set. Placing the speaker in a box lined with sheet steel prevents the speaker magnet from messing with the colors on your TV screen. Or, the speaker manufacturer may place shielding around the speaker magnet itself - much smaller and more effective.
The diagram on the right shows what happens when you place a superconductor near that magnet. The field is being repelled by the superconductor because it does not allow the field to penetrate its surface. Again, the field on the other side of the superconductor is almost nil.
Expelling the Field
Suppose you don't want any field within a region of space, how can you do that?
The diagram on the left shows a blue area that is immersed within a magnetic field. Can we eliminate the field around the blue area?
The diagram on the right shows an iron box around that region. This causes the field to travel within the walls of the box and not penetrate the inside of it. This is what is done with sensitive electronic equipment that might be affected by stray magnetic fields. They place a shield like a box or a can around the area. A good material to use is called mu-metal. An example would be oscilloscopes, that are like TV screens, where a line of electrons 'paint' the image onto the screen. Since magnetic fields affect the line of electrons, there needs to be no external field around the scope tube. A mu-metal shield, like a can, is slipped over the sides of the tube.
Containing the Field
Suppose you want to contain the magnetic field within an area and not allow it to spread out and affect other devices or your TV or other things. What can you do?
The diagram on the left shows a blue area that is again immersed within a magnetic field. We want to contain the field around the magnet and not let it spread all over the place.
The diagram on the right shows an iron box around the magnet, containing its magnetic field. The field travels within the walls of the box and do not penetrate outside of it. This is what is done when shipping magnets by air, where the field outside the shipping carton must be below a specific level for the FAA to allow it to fly.
Magnetic Shielding
These iron boxes can be steel cans as well. The shape isn't really that important. The material is very important. The thickness must increase if the field is larger in order to keep the material from saturating.
Is there anything that you could place between two magnets that will neither attract nor repel the magnets, and yet they wouldn't know that the other magnet was on the other side? I don't know of anything that could do that. If you come up with something, that would be very, very interesting!
Here's a shielding experiment that makes a great science fair project!
1. The goal is to determine what can shield a magnetic field.
2. You will need a source of a magnetic field, such as an electromagnet or a strong rare-earth magnet.
3. Next, you will need a way to determine if the magnetic field has changed strength. Certainly a sure way to do this is to measure the flux using a Gaussmeter. Another method is to use a compass to see if it is still affected the same way it was before the proposed shield was put in place.
Magnetic Shielding Box
4. Finally, you will need some plates of various materials to try as shields. I would recommend: wood, Plexiglas, styrofoam, brass, copper, aluminum, steel, iron, paper, stainless steel, and any other materials you think may work.
5. Measure the strength of the magnetic field about 2' away from the magnet. Note the value of the meter or response of the compass. Keep the meter probe or compass in one position during the experiment.
6. Place the material between the magnet and the probe or compass and see what happens. Note the material and the result.
7. What you'll find is that only steel and iron will work as a shield. If it is very thin, it's effectiveness is decreased. If you place more layers of the shield between the magnet and the probe, it will be more effective (or thicker pieces of material).
References to shielding:
http://www.magnetic-shield.com/
http://www.advancemag.com
Here's a project that did the above.

A Science Fair Project
Here is a science fair project which covered four areas:
1. A Hall-Effect Gaussmeter for measuring the strength of magnetic fields
2. A selection of magnets to compare their strengths using the Gaussmeter
3. Two electromagnets, one DC and one AC, with a variable control on them, to see how their strengths are adjustable
4. Various plates of metal to see how well they shield magnetic fields (as described above)
This shows the box with the equipment inside, and the display material.
This is a close-up of the Gaussmeter equipment in the top half. The voltmeter is on the left. The circuitry is in the middle on a breadboard. The power supply is on the right.
The bottom half is a collection of various types of magnets, all under Plexiglas. The probe is at the end of the cable laying on top. By taking the probe and holding it over the various magnets, we are able to measure the strength and polarity of the magnets.
This shows the electromagnets on the left. There is a DC electromagnet at the bottom, an AC electromagnet in the middle, and a variac for controlling the voltage to them at the top. The Gaussmeter probe can also measure the strength of these electromagnets, and their polarities. (The AC electromagnet indicates both a N and a S pole, since it is switching between them 60 times a second.)
This is a collection of metal plates which can be placed over a magnet or electromagnet, and the strength of the magnetic field on the top side of the plate can be measured, determining how well it shields the magnetic field. The plates are made of steel, aluminum, brass and copper.
Results of the shielding experiment were:
Gauss readings | ||||
Distance between probe and electromagnet | ||||
Material | 6 | 13 | 50 | mm |
air | 700 | 500 | 100 | |
aluminum | 700 | 500 | 100 | |
brass | 700 | 500 | 100 | |
copper | 700 | 500 | 100 | |
steel | 100 | 100 | 60 |
Magnetic shielding refers to the attempt to isolate or block the magnetic field of the MRI magnet. This can be done to prevent unwanted interference from the MRI magnet on nearby electronic devices. This is different from radiofrequency shielding, which is the attempt to prevent the unwanted interference of noise or radiofrequencies, often in order to avoid image distortion.
An example of where magnetic shielding is important would be when there are nearby devices that potentially can be susceptible to magnetic interference, such as cardiac pacemakers, or other sensitive pieces of electronic or medical equipment. It also becomes relevant when low frequency external magnetic fields are being used nearby and need to be shielded from the magnetic field or fringe field from the MRI magnet. In general, the entire environment around the MR magnet needs to be protected from the fringe and magnetic fields.
A conventional marker of high magnetic field proximity is the 5 gauss line. Additional magnetic shielding may be required to protect devices that fall within the 5 G line, particularly if this extends beyond the MRI exam room. 5 G is the threshold required to avoid potential influence on pacemakers. Nearby medical imaging scanners can be influenced by magnetic field strengths as low as 1-3 G.
The methods of magnetic shielding usually involve steel or copper placed in the walls of the magnet room. These metallic steel or copper plates capture the magnetic field based on their geometric make-up and result in a cancelation or blockade of the magnetic field. The magnetic shield essentially re-directs the magnetic field so that it protects the item being shielded. One of the relevant properties of the shield is that the magnetic field is attracted to the shielding material. The amount of material in the shield is also relevant, as the more material there is, the more magnetic field it can re-direct.
- 1. Jerrold T. Bushberg, John M. Boone. The Essential Physics of Medical Imaging. (2011) ISBN: 9780781780575
Magnetic Shielding Bag
Related Radiopaedia articles
Magnetic Shielding Metal
Imaging technology
Magnetic Shielding Material
- imaging physics
- x-ray
- x-ray in practice
- x-ray production
- high voltage generator
- cathode
- anode
- x-ray tubes
- filters
- grids
- x-ray film
- image intensifier
- digital radiography
- direct digital radiography
- digital image
- x-ray artifacts
- external foreign body artifact
- radiation units
- absorbed dose
- equivalent dose
- effective dose
- exposure
- legacy units
- radiation safety
- stochastic effect
- radiation-induced carcinogenesis
- stochastic effect
- radiation detectors
- dosimeters
- fluoroscopy
- computed tomography (CT)
- CT technology
- generations of CT scanners
- dual energy CT
- clinical applications of dual energy CT
- CT image reconstruction
- CT image quality
- CT dose
- CT artifacts
- patient-based artifacts
- blur
- physics-based artifacts
- hardware-based artifacts
- helical and multichannel artifacts
- MPR artifact
- helical and multichannel artifacts
- patient-based artifacts
- CT safety
- history of CT
- CT technology
- MRI
- MRI hardware
- coils
- magnets
- signal processing
- MRI pulse sequences (basics | abbreviations | parameters)
- diffusion weighted imaging (DWI)
- gradient echo sequences
- inversion recovery sequences
- perfusion-weighted imaging
- techniques
- derived values
- spin echo sequences
- MR angiography (and venography)
- non-contrast-enhanced MRA
- TRICKS
- non-contrast-enhanced MRA
- MR spectroscopy (MRS)
- 2-hydroxyglutarate peak: resonates at 2.25 ppm
- alanine peak: resonates at 1.48 ppm
- choline peak: resonates at 3.2 ppm
- citrate peak: resonates at 2.6 ppm
- creatine peak: resonates at 3.0 ppm
- functional MRI (fMRI)
- gamma-aminobutyric acid (GABA) peak: resonates at 2.2-2.4 ppm
- glutamine-glutamate peak: resonates at 2.2-2.4 ppm
- lactate peak: resonates at 1.3 ppm
- lipids peak: resonates at 1.3 ppm
- myoinositol peak: resonates at 3.5 ppm
- N-acetylaspartate (NAA) peak: resonates at 2.0 ppm
- propylene glycol peak: resonates at 1.13 ppm
- MRI artifacts
- MRI hardware and room shielding
- MRI software
- slice-overlap artifact a.k.a. cross-talk artifact
- patient and physiologic motion
- tissue heterogeneity and foreign bodies
- magnetic susceptibility artifact
- blooming artifact
- magnetic susceptibility artifact
- Fourier transform and Nyquist sampling theorem
- MRI contrast agents
- intravascular (blood pool) MRI contrast agents
- hepatobiliary MRI contrast agents
- extracellular MRI contrast agents
- contrast media safety
- MRI safety
- MRI hardware
- ultrasound
- transducers
- frame averaging (frame persistence)
- ultrasound image resolution
- imaging modes and display
- pulse-echo imaging
- Doppler imaging
- B flow
- color box
- pulse repetition frequency and scale
- color write priority
- packet size (dwell time)
- elastography
- scanning modes
- 2D ultrasound
- 4D ultrasound
- M-mode
- ultrasound artifacts
- reverberation artifact
- comet tail artifact
- hardware-related artifacts
- Doppler artifacts
- tissue vibration
- spectral broadening
- blooming
- motion (flash) artifact
- acoustic streaming
- reverberation artifact
- biological effects of ultrasound
- transducers
- nuclear medicine
- nuclear medicine physics
- nuclide
- isomer
- photopeak
- nuclide
- detectors
- scintillation detectors (gamma camera)
- emission tomography
- radiopharmaceuticals
- fundamentals of radiopharmaceuticals
- radiopharmaceutical labeling
- radiopharmaceutical production
- nuclear reactor produced radionuclides
- cyclotron produced radionuclides
- radiation detection
- dosimetry
- specific agents
- carbon-11
- chromium-51
- fluorine agents
- gallium agents
- Ga-67 citrate
- Ga-68
- iodine agents
- I-123
- I-123 iodide
- I-123 ortho-iodohippurate
- MIBG scans
- I-123 MIBG
- I-131 MIBG
- I-123
- indium agents
- In-111 Prostascint
- krypton-81m
- nitrogen-13
- oxygen-15
- phosphorus-32
- selenium-75
- technetium agents
- Tc-99m HMPAO
- Tc-99m mercaptoacetyltriglycine
- Tc-99m sulfur colloid (oral)
- xenon agents
- in vivo therapeutic agents
- nuclear medicine physics
- emerging methods in medical imaging
- radiography
- phase-contrast imaging
- CT
- deep-learning reconstruction
- ultrasound
- ultrafast Doppler imaging
- ultrasound localization microscopy
- MRI
- nuclear medicine
- total body PET system
- immuno-PET
- miscellaneous
- radiography
Magnetic Shielding Material
Promoted articles (advertising)
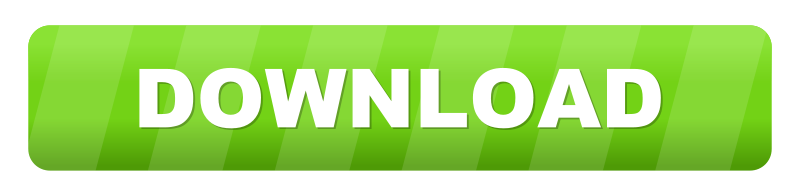